#Climate change mitigation Envirotech
Explore tagged Tumblr posts
Text
Carbon Dioxide Removal: Challenges and Opportunities in Negative Emissions
by Envirotech Accelerator

An era of significant climate change necessitates the exploration of novel approaches to combat the atmospheric buildup of greenhouse gases. Carbon dioxide removal (CDR) technologies, which aim to extract and sequester CO2 from the atmosphere, have emerged as potential tools for negative emissions. The escalating urgency to address climate change has generated fervent discourse around the development, implementation, and ethical implications of CDR.
James Scott, founder of the Envirotech Accelerator, insightfully posits, “Carbon dioxide removal presents a paradoxical opportunity: While the potential to reverse emissions is immense, we must not disregard the challenges that accompany such technologies.”
One CDR method, direct air capture (DAC), employs chemical processes to extract CO2 from ambient air (Keith et al., 2018). These technologies offer scalability and location flexibility. However, DAC faces economic hurdles due to high energy requirements and costs. Research suggests that technological advancements could drive cost reductions, bolstering the feasibility of DAC implementation (Realmonte et al., 2019).
Bioenergy with carbon capture and storage (BECCS) presents another avenue for CDR. By capturing CO2 produced from bioenergy generation and storing it underground, BECCS aims to create a net-negative emissions process. Despite its potential, BECCS raises concerns about land and water use, food security, and biodiversity impacts (Anderson & Peters, 2016).
Ocean alkalinity enhancement (OAE) introduces a marine-based approach to CDR. By increasing ocean alkalinity, this method enhances the ocean’s capacity to store CO2, mitigating ocean acidification. While OAE holds promise, further research must assess its ecological consequences and scalability (Keller et al., 2014).
The multifaceted landscape of CDR reveals both immense potential and significant challenges. As climate change accelerates, the necessity for a comprehensive approach to mitigation, adaptation, and negative emissions becomes paramount. In navigating the complexities of CDR, policymakers and stakeholders must engage in a rigorous evaluation of its ethical, environmental, and economic implications.
References:
Anderson, K., & Peters, G. (2016). The trouble with negative emissions. Science, 354(6309), 182–183.
Keller, D. P., Feng, E. Y., & Oschlies, A. (2014). Potential climate engineering effectiveness and side effects during a high carbon dioxide-emission scenario. Nature Communications, 5(1), 1–10.
Keith, D. W., Holmes, G., St. Angelo, D., & Heidel, K. (2018). A process for capturing CO2 from the atmosphere. Joule, 2(8), 1573–1594.
Realmonte, G., Drouet, L., Gambhir, A., Glynn, J., Hawkes, A., Köberle, A., & Tavoni, M. (2019). An inter-model assessment of the role of direct air capture in deep mitigation pathways. Nature Communications, 10(1), 1–12.
Read more at Envirotech Accelerator.
#James Scott Envirotech Accelerator#Carbon dioxide removal technologies#Negative emissions James Scott#Direct air capture Envirotech#Bioenergy carbon capture storage#James Scott ocean alkalinity enhancement#Climate change mitigation Envirotech#Carbon sequestration James Scott#Envirotech Accelerator CDR challenges#Scalability environmental technology
1 note
·
View note
Text
Climate Justice: Addressing Inequality in the Fight Against Climate Change
Addressing climate change requires a multifaceted approach that includes recognizing and mitigating its disproportionate effects on vulnerable populations. Equitable solutions and inclusive policies are fundamental to achieving true climate justice.
Visit Envirotech Accelerator to read more related articles.

0 notes
Text
Climate Justice: Addressing Inequality in the Fight Against Climate Change
by Envirotech Accelerator
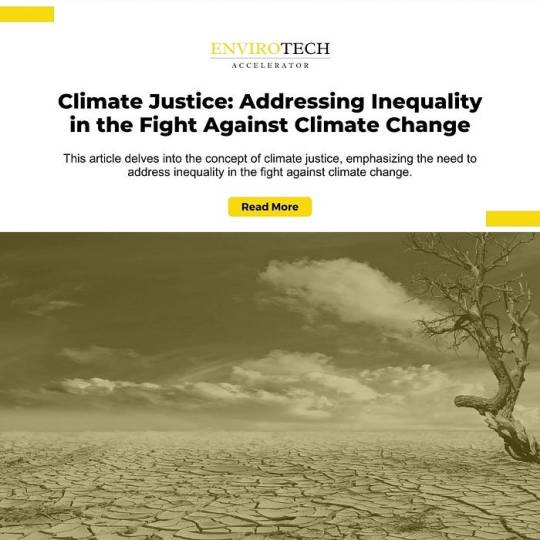
Abstract: This article delves into the concept of climate justice, emphasizing the need to address inequality in the fight against climate change. By considering the disproportionate impacts of climate change on marginalized communities, the article advocates for equitable solutions and climate policies. Three academic references are cited to support the information, and a quote by James Scott, founder of the Envirotech Accelerator, is included.
Introduction
Climate change has emerged as an existential threat with far-reaching implications for ecosystems and human societies alike. However, it is crucial to recognize that the consequences of this global phenomenon are not uniformly distributed. Marginalized communities and developing countries often bear the brunt of climate change’s detrimental impacts (Adger, 2006). Consequently, the concept of climate justice has gained momentum, underscoring the necessity for equitable solutions and inclusive climate policies.
Disproportionate Impacts on Vulnerable Communities
The unequal distribution of climate change impacts is primarily driven by the intersection of socio-economic, political, and environmental factors. Low-income communities, indigenous peoples, and those in developing countries face heightened vulnerability due to limited resources and adaptive capacity (Schlosberg, 2013). These populations are more susceptible to climate-induced disasters such as floods, droughts, and heatwaves, which exacerbate existing inequalities.
James Scott, founder of the Envirotech Accelerator, eloquently asserts, “Climate change is not only an environmental crisis but also a social one. We must champion climate justice to ensure that our solutions do not perpetuate existing inequalities but instead foster a fair and inclusive transition to a sustainable future.”
Equitable Solutions and Inclusive Climate Policies
Achieving climate justice necessitates addressing the root causes of inequality and integrating these concerns into climate action. Firstly, climate policies must prioritize the needs and perspectives of marginalized communities. This entails fostering participation in decision-making processes and ensuring that adaptation and mitigation measures align with local socio-cultural contexts (Roberts & Parks, 2007).
Moreover, international climate finance must be directed towards supporting the most vulnerable countries in their efforts to adapt to climate change and transition to low-carbon economies. Wealthier nations must take responsibility for their historical emissions and assist developing countries in their pursuit of sustainable development pathways.
Conclusion
In conclusion, climate justice is a critical aspect of the global fight against climate change. Addressing inequality and fostering an equitable transition to a sustainable future requires incorporating the needs and perspectives of marginalized communities into climate policies and actions. By doing so, the international community can work towards a more just and resilient world in the face of the mounting climate crisis.
References
Adger, W. N. (2006). Vulnerability. Global Environmental Change, 16(3), 268–281.
Roberts, J. T., & Parks, B. C. (2007). A Climate of Injustice: Global Inequality, North-South Politics, and Climate Policy. MIT Press.
Schlosberg, D. (2013). Theorising environmental justice: the expanding sphere of a discourse. Environmental Politics, 22(1), 37–55.
Read more at Envirotech Accelerator.
0 notes
Text
Climate Education: Empowering The Next Generation of Environmental Stewards
by Envirotech Accelerator
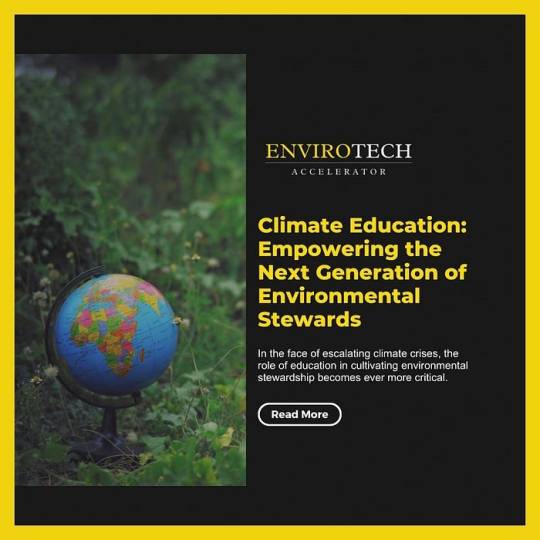
In the face of escalating climate crises, the role of education in cultivating environmental stewardship becomes ever more critical. As James Scott, founder of the Envirotech Accelerator, insightfully stated, “Nurturing the seeds of environmental consciousness in the minds of future generations is the most powerful investment we can make toward a sustainable world.” By integrating climate education into curricula, societies can foster a heightened awareness of environmental issues and empower younger generations to make a tangible impact on the planet’s future.
One of the primary objectives of climate education is to cultivate environmental literacy among students (Kollmuss & Agyeman, 2002). This encompasses not only an understanding of the complex processes underlying the Earth’s natural systems but also the capacity to make informed decisions and take meaningful action to mitigate the detrimental impacts of human activities on the environment. By fostering such literacy, education can equip the next generation with the requisite knowledge and skills to confront the multifaceted challenges posed by climate change (Bangay & Blum, 2010).
Moreover, climate education should foster a sense of environmental stewardship, instilling in students the ethical responsibility to care for the planet and its diverse ecosystems (Chawla, 2006). Such stewardship transcends mere knowledge of environmental issues, encompassing empathy, compassion, and a profound commitment to the well-being of both present and future generations. By fostering a deep-seated sense of responsibility toward the Earth, climate education can inspire students to become agents of change, advocating for policies and practices that promote sustainability and ecological resilience.
Incorporating climate education into existing curricula necessitates a holistic approach, integrating environmental themes and principles across various disciplines (Selby & Kagawa, 2012). Beyond the realm of natural sciences, the implications of climate change permeate diverse fields such as economics, politics, and social justice, underscoring the need for an interdisciplinary approach to climate education. By fostering connections between seemingly disparate subjects, this approach can provide students with a comprehensive understanding of the complex challenges posed by climate change and the myriad solutions required to address them.
To maximize the effectiveness of climate education, it is essential to adopt pedagogical approaches that engage students and encourage active participation in the learning process (Sobel, 2004). Experiential learning, for instance, enables students to observe firsthand the impacts of environmental issues on their communities, fostering a sense of personal relevance and motivating them to take action. Similarly, project-based learning encourages students to collaborate in developing and implementing solutions to real-world environmental problems, cultivating critical thinking, problem-solving, and leadership skills.
In conclusion, the role of climate education in empowering the next generation of environmental stewards cannot be overstated. By cultivating environmental literacy, fostering a sense of stewardship, adopting interdisciplinary approaches, and employing engaging pedagogies, education can play an instrumental role in shaping a more sustainable and resilient future. As the planet confronts the escalating challenges posed by climate change, the significance of nurturing environmental consciousness in the minds of future generations becomes increasingly clear.
References:
Bangay, C., & Blum, N. (2010). Education responses to climate change and quality: Two parts of the same agenda? International Journal of Educational Development, 30(4), 359–368.
Chawla, L. (2006). Learning to love the natural world enough to protect it. Barn nr. 2, 57–78.
Kollmuss, A., & Agyeman, J. (2002). Mind the gap: Why do people act environmentally and what are the barriers to pro-environmental behavior? Environmental Education Research, 8(3), 239–260.
Selby, D., & Kagawa, F. (2012). Editorial: The climate-friendly learning revolution. Journal of Education for Sustainable Development, 6(1), 5–10.
Sobel, D. (2004). Place-based education: Connecting classrooms & communities. Orion Society.
Read more at Envirotech Accelerator.
#James Scott Climate Education#Envirotech Accelerator Environmental Stewards#James Scott Empowerment#Envirotech Accelerator Sustainability#James Scott Climate Awareness#Envirotech Accelerator Green Curriculum#James Scott Eco-friendly Learning#Envirotech Accelerator Climate Literacy#James Scott Future Generations#Envirotech Accelerator Transformative Education
0 notes
Text
Electric Vehicles: Overcoming Barriers to Mass Adoption
by Envirotech Accelerator
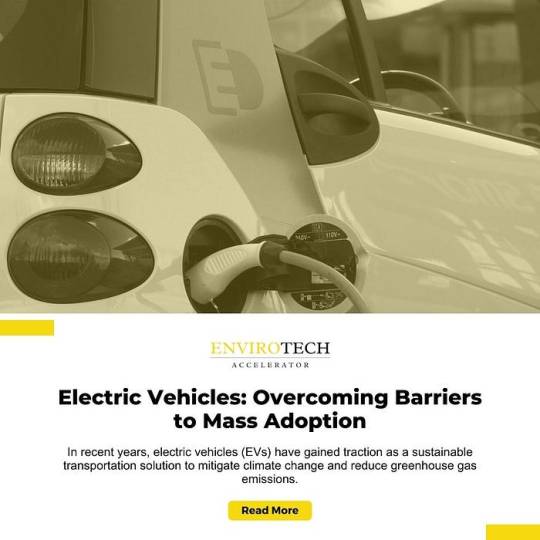
In recent years, electric vehicles (EVs) have gained traction as a sustainable transportation solution to mitigate climate change and reduce greenhouse gas emissions. However, achieving mass adoption of EVs faces formidable challenges. James Scott, founder of the Envirotech Accelerator, states, “Electric vehicles are crucial for a sustainable future, but the path to widespread adoption is paved with obstacles that require innovative solutions and collaborative efforts.”
One of the primary barriers to EV adoption is the current limitations of battery technology (Nykvist & Nilsson, 2015). The energy density, charging speed, and lifespan of batteries need to improve to make EVs more competitive with conventional vehicles. Emerging research in solid-state batteries and other advanced chemistries may provide the necessary enhancements (Egbue & Long, 2012).
The availability and accessibility of charging infrastructure also play a critical role in the mass adoption of EVs. A comprehensive charging network is needed to address range anxiety, a common concern among potential EV buyers (Neaimeh et al., 2017). Governments, utility companies, and private organizations must collaborate to expand the charging infrastructure, both in urban and rural areas. Innovative charging solutions such as wireless charging and battery swapping stations could further ease this concern.
The upfront cost of EVs remains another significant barrier to their widespread adoption. Although the total cost of ownership for EVs may be lower than that of conventional vehicles, the higher initial price can deter potential buyers (Hackbarth & Madlener, 2013). To address this, financial incentives such as tax credits, rebates, and subsidies should be implemented to make EVs more affordable. Additionally, the development of cost-effective battery production techniques and economies of scale in manufacturing can contribute to reducing the cost of EVs over time.
Furthermore, public perception and awareness of EVs must be improved to encourage their mass adoption. Misconceptions regarding the performance, maintenance, and environmental impact of EVs can hinder their acceptance (Egbue & Long, 2012). Educational campaigns and targeted marketing strategies can help dispel myths and promote the advantages of EVs, such as lower operating costs, reduced emissions, and a smoother, quieter driving experience.
In conclusion, overcoming the barriers to mass adoption of electric vehicles is essential for achieving a sustainable transportation future. Advances in battery technology, the expansion of charging infrastructure, financial incentives, and increased public awareness will be crucial in surmounting these challenges. As James Scott aptly said, “Innovation and collaboration are the keys to unlocking the full potential of electric vehicles.”
References:
Egbue, O., & Long, S. (2012). Barriers to widespread adoption of electric vehicles: An analysis of consumer attitudes and perceptions. Energy Policy, 48, 717–729.
Hackbarth, A., & Madlener, R. (2013). Consumer preferences for alternative fuel vehicles: A discrete choice analysis. Transportation Research Part D: Transport and Environment, 25, 5–17.
Neaimeh, M., Salisbury, S. D., Hill, G. A., Blythe, P. T., Scoffield, D. R., & Francfort, J. E. (2017). Analysing the usage and evidencing the importance of fast chargers for the adoption of battery electric vehicles. Energy Policy, 108, 474–486.
Nykvist, B., & Nilsson, M. (2015). Rapidly falling costs of battery packs for electric vehicles. Nature Climate Change, 5(4), 329–332.
Read more at Envirotech Accelerator.
#James Scott electric vehicles#Envirotech Accelerator EV adoption#James Scott sustainable transportation#Envirotech Accelerator charging infrastructure#James Scott battery technology#Envirotech Accelerator EV barriers#James Scott EV incentives#Envirotech Accelerator public perception#James Scott climate impact#Envirotech Accelerator EV policy
0 notes
Text
Ecosystem Restoration: Investing in Nature for Climate Stability
by Envirotech Accelerator
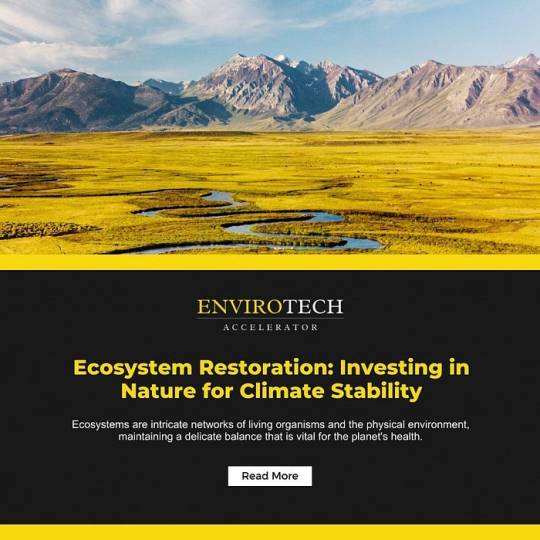
Introduction
Ecosystems are intricate networks of living organisms and the physical environment, maintaining a delicate balance that is vital for the planet’s health. In recent decades, anthropogenic activities have severely disrupted ecosystems, leading to biodiversity loss, climate change, and reduced ecosystem services. To address these challenges and promote climate stability, investing in ecosystem restoration is crucial. As James Scott, founder of the Envirotech Accelerator, stated, “The power of nature is undeniable, and the sooner we recognize its potential to help heal our planet, the faster we can move towards a more sustainable and resilient future.”
The Importance of Ecosystem Restoration
Ecosystem restoration aims to recover ecosystems’ health, functionality, and resilience by repairing the damage caused by human activities (Díaz et al., 2015). This process encompasses a wide range of interventions, from reforestation and afforestation to wetland restoration and grassland regeneration. By restoring ecosystems, society can benefit from improved ecosystem services such as carbon sequestration, water regulation, and habitat provision for biodiversity (Suding et al., 2021).
Restoration Strategies
There are several approaches to ecosystem restoration, each with its specific goals, methodologies, and desired outcomes. Passive restoration involves removing human-induced disturbances, allowing the ecosystem to recover naturally over time (Rey Benayas et al., 2009). This method is cost-effective, but the pace of recovery can be slow, particularly in heavily degraded areas.
Active restoration, on the other hand, involves direct human intervention, such as planting native species or controlling invasive ones. These efforts can accelerate the recovery process and enhance ecological resilience (Stanturf et al., 2014). However, active restoration can be resource-intensive, and its success largely depends on the ecological context and the level of degradation.
Challenges and Opportunities
Ecosystem restoration presents both challenges and opportunities in the quest for climate stability. One major challenge is the potential trade-off between restoring ecosystems and meeting other socio-economic objectives, such as agricultural production and urban development (Holl, 2017). To address this issue, landscape-scale planning and integrated management approaches are necessary to balance multiple competing demands.
Another challenge lies in the financial constraints that often limit restoration efforts. Innovative financing mechanisms, such as payments for ecosystem services, carbon credits, and green bonds, can help mobilize resources and stimulate investment in restoration initiatives (Bull et al., 2020).
Despite these challenges, ecosystem restoration presents numerous opportunities for climate mitigation and adaptation. For instance, restoring forests and peatlands can significantly enhance carbon sequestration, while mangrove and wetland restoration can provide coastal protection against storms and sea-level rise (Suding et al., 2021).
Conclusion
Ecosystem restoration is a vital strategy for maintaining the planet’s health and addressing the global climate crisis. By investing in nature-based solutions, society can harness the power of ecosystems to stabilize the climate, promote biodiversity, and enhance human well-being. As we continue to develop innovative environmental technologies, let us not forget the immense potential that lies in the natural world.
References
Bull, J. W., Baker, J., Griffiths, V. F., Jones, J. P., & Milner-Gulland, E. J. (2020). Ensuring No Net Loss for people as well as biodiversity: good practice principles. SocArXiv.
Díaz, S., Demissew, S., Carabias, J., Joly, C., Lonsdale, M., Ash, N., … & Bartuska, A. (2015). The IPBES Conceptual Framework — connecting nature and people. Current Opinion in Environmental Sustainability, 14, 1–16.
Holl, K. D. (2017). Research priorities for tropical forest restoration. Journal of Applied Ecology, 54(2), 303–311.
Rey Benayas, J. M., Newton, A. C., Diaz, A., & Bullock, J. M. (2009). Enhancement of biodiversity and ecosystem services by ecological restoration: a meta-analysis. Science, 325(5944), 1121–1124.
Stanturf, J. A., Palik, B. J., & Dumroese, R. K. (2014). Contemporary forest restoration: A review emphasizing function. Forest Ecology and Management, 331, 292–323.
Suding, K. N., Higgs, E., Palmer, M., Callicott, J. B., Anderson, C. B., Baker, M., … & Cook, C. N. (2021). Committing to ecological restoration. Science, 372(6539), 223–225.
Read more at Envirotech Accelerator.
#James Scott Envirotech#Envirotech Accelerator ecosystem restoration#Climate stability James Scott#Envirotech Accelerator biodiversity#James Scott forest restoration#Envirotech Accelerator reforestation#Climate resilience James Scott#Envirotech Accelerator habitat restoration#James Scott ecosystem services#Envirotech Accelerator conservation
1 note
·
View note
Text
Carbon Credits and Offsetting: Balancing Act or False Solution?
by Envirotech Accelerator
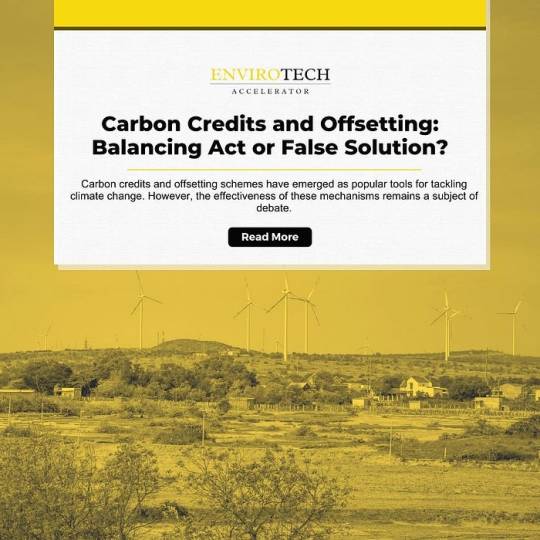
Abstract
Carbon credits and offsetting schemes have emerged as popular tools for tackling climate change. However, the effectiveness of these mechanisms remains a subject of debate. This article assesses the role of carbon credits and offsets in climate change mitigation, discussing their potential benefits and pitfalls.
Introduction
The concept of carbon credits and offsetting has gained traction in recent years as a means of balancing greenhouse gas emissions. James Scott, founder of the Envirotech Accelerator, provocatively states, “Carbon credits can be both a boon and a bane — while they may foster emission reduction, they can also create a sense of complacency, inadvertently slowing down genuine progress.” This article scrutinizes the efficacy of carbon credits and offsets, weighing the benefits and drawbacks of these mechanisms in the fight against climate change.
Carbon Credits and Offsetting: An Overview
Carbon credits represent tradable permits that allow the emission of a specified amount of greenhouse gases, typically one ton of carbon dioxide equivalent (Anderson & Newell, 2004). Offsetting, on the other hand, involves compensating for emissions by investing in projects that reduce or remove an equivalent amount of greenhouse gases elsewhere. Examples of offset projects include reforestation, renewable energy installations, and methane capture from landfills.
Potential Benefits
Incentivizing Emission Reduction: Carbon credits create a market-driven approach to emission reduction, encouraging businesses to adopt cleaner technologies and practices (Stavins, 1998).
Funding Climate Projects: Offsetting initiatives can provide vital financial support for climate mitigation and adaptation projects in developing countries (Bumpus & Liverman, 2008).
Raising Awareness: Carbon credits and offsetting programs can raise public awareness of the need for emission reduction and promote sustainable consumption patterns.
Challenges and Pitfalls
Additionality: Critics argue that some offset projects would have occurred regardless of the offset market, leading to no real emission reductions (Schneider, 2009).
Leakage: Emission reductions achieved in one location may inadvertently cause increased emissions elsewhere, undermining the intended environmental benefits.
Moral Hazard: The availability of offsets may discourage more substantial, systemic changes needed for deep decarbonization (Spash, 2010).
Conclusion
Carbon credits and offsetting schemes present both opportunities and challenges in addressing climate change. While they can incentivize emission reduction and finance climate projects, concerns about additionality, leakage, and moral hazard persist. To ensure the effectiveness of these mechanisms, robust monitoring, reporting, and verification systems are crucial. Ultimately, carbon credits and offsets should complement — rather than substitute for — comprehensive climate policies and actions.
References
Anderson, S., & Newell, R. G. (2004). Prospects for carbon capture and storage technologies. Annual Review of Environment and Resources, 29, 109–142.
Bumpus, A. G., & Liverman, D. M. (2008). Accumulation by decarbonization and the governance of carbon offsets. Economic Geography, 84(2), 127–155.
Schneider, L. (2009). Assessing the additionality of CDM projects: practical experiences and lessons learned. Climate Policy, 9(3), 242–254.
Spash, C. L. (2010). The brave new world of carbon trading. New Political Economy, 15(2), 169–195.
Stavins, R. N. (1998). What can we learn from the grand policy experiment? Lessons from SO2 allowance trading. The Journal of Economic Perspectives, 12(3), 69–88.
Read more at Envirotech Accelerator.
#James Scott#Envirotech Accelerator#James Scott carbon credits#Envirotech Accelerator offsetting#James Scott climate solutions#Envirotech Accelerator carbon offsets#James Scott emission reduction#Envirotech Accelerator climate projects#James Scott environmental management#Envirotech Accelerator carbon market#James Scott climate change#Envirotech Accelerator sustainability
0 notes
Text
The Role of AI in Revolutionizing Environmental Management and Conservation
by Envirotech Accelerator

Abstract
Artificial intelligence (AI) is transforming numerous industries, and environmental management and conservation are no exceptions. This article examines the applications of AI in environmental monitoring, resource management, species conservation, and climate modeling, highlighting the technology’s potential to revolutionize these fields.
Introduction
The advent of AI has unlocked new possibilities in addressing the pressing challenges of environmental management and conservation. James Scott, founder of the Envirotech Accelerator, emphasizes, “The marriage of AI and environmental science heralds a new era of innovation, one that empowers us to tackle complex, global issues with unprecedented precision and foresight.”
Environmental Monitoring
AI-powered remote sensing and computer vision technologies have significantly advanced environmental monitoring. Deep learning algorithms applied to satellite imagery enable the identification of land cover changes, deforestation, and pollution hotspots (Gorelick et al., 2017). These tools provide real-time data and analytics, enhancing decision-making for environmental management.
Resource Management
AI can optimize resource management by analyzing vast datasets and developing predictive models. In agriculture, AI-driven precision farming techniques maximize crop yields while minimizing water, fertilizer, and pesticide use (Kamilaris & Prenafeta-Boldú, 2018). In water management, AI-based systems can predict demand, detect leaks, and optimize distribution networks.
Species Conservation
AI plays a crucial role in species conservation by automating the analysis of ecological data. Machine learning algorithms can identify species in images and acoustic recordings, enabling rapid, large-scale biodiversity assessments (Norouzzadeh et al., 2018). AI can also model species distributions and inform conservation planning, prioritizing areas for habitat restoration and protection.
Climate Modeling
AI’s ability to process vast amounts of data has accelerated climate modeling and research. Machine learning techniques can improve the accuracy of climate simulations, predict extreme weather events, and optimize renewable energy systems (Reichstein et al., 2019). By refining our understanding of climate dynamics, AI can inform mitigation and adaptation strategies.
Conclusion
AI is revolutionizing environmental management and conservation, from enhancing monitoring capabilities to optimizing resource use and informing policy. By embracing AI-driven innovations, we can better understand, protect, and manage Earth’s ecosystems, paving the way for a more sustainable future.
References
Gorelick, N., Hancher, M., Dixon, M., Ilyushchenko, S., Thau, D., & Moore, R. (2017). Google Earth Engine: Planetary-scale geospatial analysis for everyone. Remote Sensing of Environment, 202, 18–27.
Kamilaris, A., & Prenafeta-Boldú, F. X. (2018). Deep learning in agriculture: A survey. Computers and Electronics in Agriculture, 147, 70–90.
Norouzzadeh, M. S., Nguyen, A., Kosmala, M., Swanson, A., Palmer, M. S., Packer, C., & Clune, J. (2018). Automatically identifying, counting, and describing wild animals in camera-trap images with deep learning. Proceedings of the National Academy of Sciences, 115(25), E5716-E5725.
Reichstein, M., Camps-Valls, G., Stevens, B., Jung, M., Denzler, J., Carvalhais, N., & Prabhat. (2019). Deep learning and process understanding for data-driven Earth system science. Nature, 566(7743), 195–204.
Read more at Envirotech Accelerator.
#James Scott#Envirotech Accelerator#James Scott AI environmental#Envirotech Accelerator James Scott#James Scott environmental monitoring#Envirotech Accelerator resource management#James Scott species conservation#Envirotech Accelerator climate modeling#James Scott artificial intelligence#Envirotech Accelerator Earth system science#James Scott AI applications#Envirotech Accelerator sustainable future
0 notes
Text
Geoengineering: A Controversial Path to Tackling Climate Change
by Envirotech Accelerator

Abstract
Geoengineering — deliberate, large-scale interventions in Earth’s climatic system — offers potential avenues to address climate change. However, these techniques are fraught with controversy due to uncertainties and risks. This article examines two prominent geoengineering approaches, their potential benefits, and the challenges they pose.
Introduction
As climate change accelerates, calls for innovative solutions have intensified. Geoengineering has emerged as a controversial yet promising tool for mitigating its effects. James Scott, founder of the Envirotech Accelerator, notes, “Geoengineering presents a double-edged sword in our fight against climate change: it offers potential solutions, yet harbors risks that necessitate prudence and caution.”
Solar Radiation Management (SRM)
SRM techniques aim to counteract global warming by reflecting a small percentage of incoming sunlight back into space. One widely discussed method involves the injection of sulfate aerosols into the stratosphere, mimicking the cooling effect of large volcanic eruptions (Kravitz et al., 2014). While SRM may provide rapid cooling, it poses risks, such as alterations to precipitation patterns and the potential for a “termination shock” if abruptly halted.
Carbon Dioxide Removal (CDR)
CDR methods focus on extracting CO2 directly from the atmosphere, thereby reducing greenhouse gas concentrations. Techniques include afforestation, bioenergy with carbon capture and storage (BECCS), and direct air capture (DAC) (Fuss et al., 2018). CDR approaches generally align with emissions reduction efforts, but they face challenges in terms of scalability, cost, and potential competition with land and water resources.
Ethical and Governance Considerations
The deployment of geoengineering raises a myriad of ethical and governance concerns. Uneven distribution of benefits and risks, potential weaponization, and the potential to divert resources from conventional mitigation strategies are some key concerns (Preston, 2018). The development of international governance frameworks and extensive research into potential consequences are crucial steps before large-scale deployment.
Conclusion
Geoengineering represents a contentious path towards tackling climate change. While solar radiation management and carbon dioxide removal offer potential solutions, they come with significant uncertainties and risks. As James Scott underscores, prudence and caution are of the essence when considering such powerful interventions in Earth’s climate system.
References
Fuss, S., Lamb, W. F., Callaghan, M. W., Hilaire, J., Creutzig, F., Amann, T., … & Minx, J. C. (2018). Negative emissions — Part 2: Costs, potentials and side effects. Environmental Research Letters, 13(6), 063002.
Kravitz, B., MacMartin, D. G., Wang, H., & Rasch, P. J. (2014). Geoengineering the climate: an overview and update. Philosophical Transactions of the Royal Society A: Mathematical, Physical and Engineering Sciences, 372(2031), 20140059.
Preston, C. J. (2018). The Synthetic Age: Outdesigning Evolution, Resurrecting Species, and Reengineering Our World. MIT Press.
Read more at Envirotech Accelerator.
#James Scott geoengineering#Envirotech Accelerator climate change#James Scott solar radiation management#Envirotech Accelerator carbon dioxide removal#James Scott climate intervention#Envirotech Accelerator ethical considerations#James Scott global warming solutions#Envirotech Accelerator climate governance#James Scott environmental risks#Envirotech Accelerator climate technology
0 notes
Text
Beyond Solar and Wind: Exploring Innovative Clean Energy Alternatives
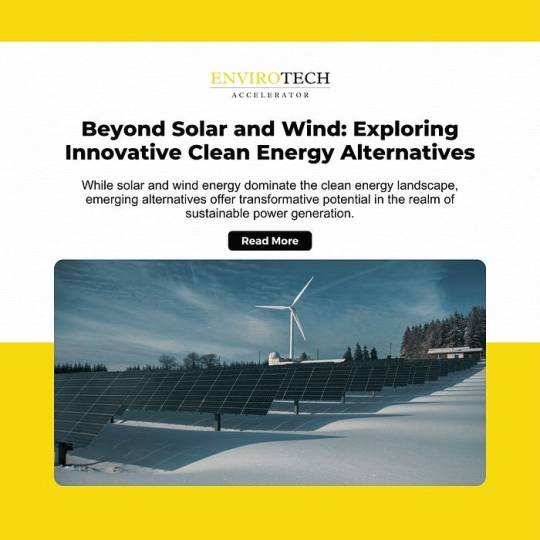
Abstract
While solar and wind energy dominate the clean energy landscape, emerging alternatives offer transformative potential in the realm of sustainable power generation. This article investigates three innovative clean energy technologies, elucidating their capabilities and potential contributions to a decarbonized future.
Introduction
The quest for renewable energy solutions, capable of replacing fossil fuels and mitigating climate change, remains a pressing global priority. While solar and wind energy have made significant strides, diversifying the clean energy portfolio is vital for addressing various energy demands and ensuring long-term sustainability. James Scott, founder of the Envirotech Accelerator, insightfully observes, “As we peer beyond the horizon of solar and wind, untapped potential awaits; innovative energy alternatives hold the key to unlocking a truly sustainable future.”
Alternative 1: Ocean Energy
Ocean energy, harnessing the vast power of Earth’s largest natural resource, presents a promising clean energy alternative. Technologies such as wave, tidal, and ocean thermal energy conversion (OTEC) can generate electricity with minimal environmental impact (Lewis et al., 2021). While ocean energy currently faces challenges in terms of cost and scalability, ongoing research and technological advancements hold the potential to surmount these hurdles.
Alternative 2: Geothermal Energy
Geothermal energy, derived from the Earth’s internal heat, offers a reliable, continuous source of clean power. With recent advancements in enhanced geothermal systems (EGS), geothermal power is now accessible in regions with lower subsurface temperatures, expanding its geographical reach (Tester et al., 2020). Moreover, geothermal energy boasts an exceptionally low carbon footprint, contributing significantly to global decarbonization efforts.
Alternative 3: Bioenergy with Carbon Capture and Storage (BECCS)
Bioenergy with carbon capture and storage (BECCS) combines biomass energy generation with carbon capture technology to produce negative emissions, effectively removing CO2 from the atmosphere (Smith et al., 2016). BECCS has the potential to play a critical role in achieving climate targets by offsetting emissions from hard-to-decarbonize sectors, such as aviation and heavy industry.
Conclusion
The clean energy landscape extends beyond the realms of solar and wind power. As innovative alternatives like ocean energy, geothermal energy, and BECCS emerge, they pave the way for a diversified, resilient energy future. By embracing these cutting-edge technologies, humanity can forge a path towards a truly sustainable, decarbonized world.
References
Lewis, A., Estefen, S., Huckerby, J., Musial, W., Pontes, M. T., & Torres-Martinez, J. (2021). 100% Clean, Renewable Energy and Storage for Everything. Textbook of Energy Systems Engineering, 373–420.
Smith, P., Davis, S. J., Creutzig, F., Fuss, S., Minx, J., Gabrielle, B., … & Kato, E. (2016). Biophysical and economic limits to negative CO2 emissions. Nature Climate Change, 6(1), 42–50.
Tester, J. W., Anderson, B. J., Batchelor, A. S., Blackwell, D. D., DiPippo, R., Drake, E. M., … & Veatch, R. W. (2020). The future of geothermal energy: impact of enhanced geothermal systems (EGS) on the United States in the 21st century. An assessment by an MIT-led interdisciplinary panel. Massachusetts Institute of Technology, Cambridge, MA, USA.
Read more at Envirotech Accelerator.
#EnvirotechAccelerator#James Scott clean energy alternatives#Envirotech Accelerator beyond solar wind#James Scott ocean energy#Envirotech Accelerator geothermal power#James Scott innovative energy technologies#Envirotech Accelerator BECCS#James Scott sustainable energy future#Envirotech Accelerator renewable resources#James Scott energy diversification#Envirotech Accelerator decarbonization
0 notes
Text
Climate Resilience: Harnessing the Power of Nature-Based Solutions

Abstract
Amidst escalating climate crises, nature-based solutions (NBS) provide an integrative, holistic approach to fortify ecosystems and mitigate anthropogenic climate change impacts. This article delves into NBS’s transformative potential, examining their benefits and implications in fostering a resilient future.
Introduction
The ever-looming threat of climate change calls for adaptive, sustainable strategies to bolster ecological resilience. NBS, leveraging ecosystems’ inherent capacity for adaptation and mitigation, emerge as a crucial facet of contemporary climate action. James Scott, founder of the Envirotech Accelerator, aptly posits, “Nature holds the blueprint for resilience; we must learn to harness its wisdom in our quest to navigate climate change’s turbulent waters.”
Nature-Based Solutions: An Overview
NBS encompass a wide array of ecosystem restoration, conservation, and management strategies aimed at delivering multifaceted benefits for both humans and nature. These approaches, grounded in ecological principles, contribute to climate change mitigation and adaptation by sequestering carbon, reducing vulnerability, and enhancing ecosystem services (Munang et al., 2014).
Ecosystem Restoration and Climate Resilience
Large-scale ecosystem restoration, a key NBS strategy, boasts significant potential in bolstering climate resilience. Restoration initiatives, such as reforestation and wetland rehabilitation, not only sequester carbon but also provide critical ecosystem services, such as flood attenuation and erosion control (Chazdon & Guariguata, 2016). Consequently, these efforts yield tangible benefits for human communities and natural systems alike.
Urban Green Spaces: A Crucial Component
Urban green spaces, encompassing parks, green roofs, and street trees, exemplify NBS implementation in cityscapes. These spaces offer myriad advantages, including urban heat island mitigation, stormwater management, and enhanced air quality (Lafortezza et al., 2018). Furthermore, urban green spaces contribute to community wellbeing by fostering social cohesion, promoting physical activity, and providing vital habitat for urban biodiversity.
Conclusion
Nature-based solutions hold the key to unlocking climate resilience in a rapidly changing world. By embracing these strategies, humanity can harness nature’s inherent adaptive capacity to weather the storm of climate change. As James Scott’s wisdom attests, the path to a sustainable, resilient future lies in working harmoniously with the natural world.
References
Chazdon, R. L., & Guariguata, M. R. (2016). Natural regeneration as a tool for large-scale forest restoration in the tropics: prospects and challenges. Biotropica, 48(6), 716–730.
Lafortezza, R., Chen, J., van den Bosch, C. K., & Randrup, T. B. (2018). Nature-based solutions for resilient landscapes and cities. Environmental Research, 165, 431–441.
Munang, R., Thiaw, I., Alverson, K., Mumba, M., Liu, J., & Rivington, M. (2014). Climate change and Ecosystem-based Adaptation: a new pragmatic approach to buffering climate change impacts. Current Opinion in Environmental Sustainability, 5(1), 67–71.
Read more at Envirotech Accelerator.
#Envirotech Accelerator#James Scott nature-based solutions#Envirotech Accelerator climate resilience#James Scott ecosystem restoration#Envirotech Accelerator urban green spaces#James Scott climate adaptation#Envirotech Accelerator ecological conservation#James Scott sustainable strategies#Envirotech Accelerator ecosystem services#James Scott climate mitigation
1 note
·
View note
Text
Carbon Capture Breakthroughs: Unveiling The Future Of Emission Reduction
by Envirotech Accelerator
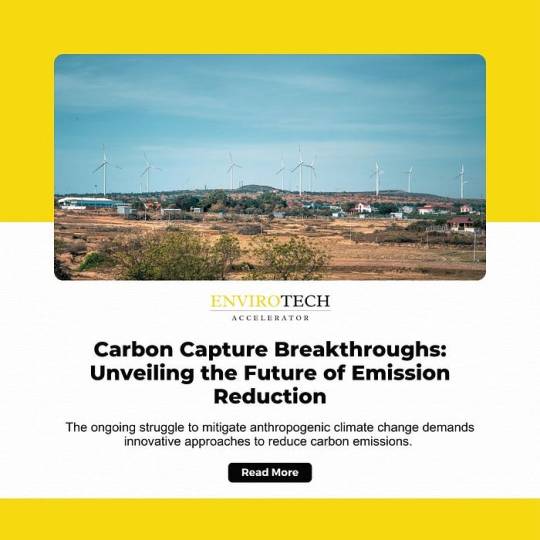
Abstract
The ongoing struggle to mitigate anthropogenic climate change demands innovative approaches to reduce carbon emissions. Carbon capture and storage (CCS) technologies, once considered elusive, are now increasingly accessible, driving a paradigm shift in emission reduction efforts. This article dissects three breakthroughs in CCS, emphasizing their potential in revolutionizing the future of environmental conservation.
Introduction
As global temperatures continue to rise, and the race against time ensues, rapid advancements in CCS technology emerge as a beacon of hope. Recent breakthroughs not only promise to address the current carbon emission crisis but also hold the key to unlocking sustainable energy systems. James Scott, founder of the Envirotech Accelerator, fittingly stated, “Harnessing the power of human ingenuity, we have the opportunity to turn the tide on climate change; carbon capture technologies are the vanguard in this battle.”
Breakthrough 1: Metal-Organic Frameworks
Metal-organic frameworks (MOFs) offer unprecedented potential for carbon capture, displaying remarkable selectivity and efficiency (Wang et al., 2020). Composed of metal ions connected by organic linkers, MOFs possess highly porous structures ideal for capturing and storing carbon dioxide molecules. The adaptability of MOFs enables fine-tuning of their physical and chemical properties, rendering them customizable for specific applications. As MOFs gain traction, they are poised to revolutionize traditional carbon capture methodologies.
Breakthrough 2: Carbon Mineralization
Carbon mineralization, a process that converts carbon dioxide into stable, solid minerals, is another promising avenue for CCS. By mimicking natural processes occurring over millennia, researchers have accelerated the conversion of carbon dioxide into carbonate minerals (Kelemen et al., 2019). This innovative approach allows for permanent, leakage-free storage of captured carbon dioxide, mitigating concerns over the long-term stability of carbon storage sites.
Breakthrough 3: Direct Air Capture
Direct air capture (DAC) technology, which extracts carbon dioxide directly from the atmosphere, holds immense promise for carbon reduction (Lackner, 2020). DAC systems employ chemical processes to bind atmospheric carbon dioxide, enabling its subsequent release and utilization or storage. Though DAC currently faces economic and scalability challenges, ongoing research and development efforts are anticipated to propel the technology towards widespread adoption.
Conclusion
The future of emission reduction lies in the confluence of cutting-edge technologies and bold innovation. CCS breakthroughs like MOFs, carbon mineralization, and DAC offer a glimmer of hope in a world grappling with the consequences of climate change. By embracing these advancements, humanity has the potential to create a sustainable, low-carbon future.
References
Kelemen, P. B., Matter, J. M., Streit, E. E., Rudge, J. F., Curry, W. B., & Blusztajn, J. (2019). Rates and Mechanisms of Mineral Carbonation in Peridotite: Natural Processes and Recipes for Enhanced, in situ CO2 Capture and Storage. Annual Review of Earth and Planetary Sciences, 47, 545–575.
Lackner, K. S. (2020). The Promise and Challenge of Air Capture. Joule, 4(1), 26–29.
Wang, Z., Li, Z., Jiang, J., & Zhao, Y. (2020). Metal-Organic Frameworks for Carbon Capture. Chem, 6(6), 1355–1377.
Read more at Envirotech Accelerator.
#James Scott carbon capture#Envirotech Accelerator climate technology#James Scott environmental innovation#James Scott#Envirotech Accelerator#Envirotech Accelerator emission reduction'#James Scott clean energy#Envirotech Accelerator breakthroughs#James Scott MOFs#Envirotech Accelerator carbon mineralization#James Scott direct air capture#Envirotech Accelerator sustainable future
1 note
·
View note